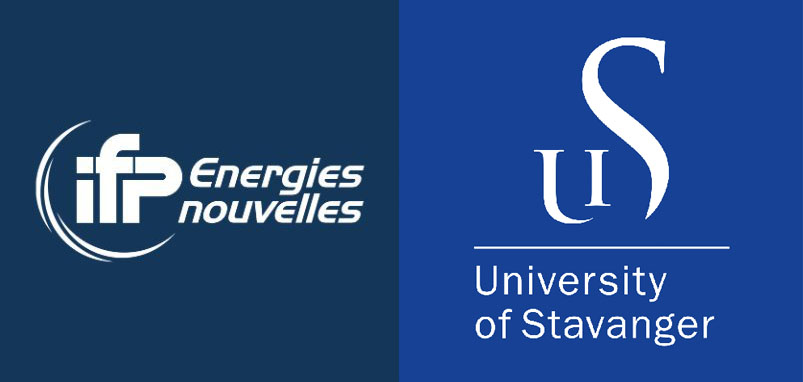
Research Part
Work package 4 aims to increase the scientific knowledge of IG/FORTH in underground hydrogen storage and focus on the potential sites of underground storage of H2 and the potential synergies or comapetition of other uses of the underground space of other gases such as CO2.
The research work is divided in 3 main research tasks as follows:
Task 4.1 IDENTIFICATION OF SUITABLE GEOLOGICAL FORMATIONS FOR HYDROGEN STORAGE
The aim of 4.1 is to identify of suitable geological formations for large scale hydrogen storage.
Research Task 4.1.1: Analysis of geological and reservoir conditions of the underground storage of hydrogen (UiS(L), ALL PARTNERS)
The reservoir tightness is one of the most important geological and reservoir conditions when taking the appropriate porosity and permeability of rocks building underground storage sites into account. The aim of this task is to identify important elements and assess their importance and impact in determining the suitability of a particular geological structure for hydrogen storage in porous media. The geological and reservoir conditions for underground hydrogen storage in salt caverns, deep aquifers and oil-/gas reservoirs will be examined in detail.
Research Task 4.1.2: Potential synergies of underground gas storage sites and Lessons Learnt (UiS(L), ALL PARTNERS)
A specific rock formation may be suitable for various forms of underground gas storage. There is a competition among H2, CH4 and CO2 regarding the use of underground space. Various factors should be taken into account, including: reservoir depth and geology, energy density, technology efficiency, sustainable underground space planning, and others. Assigning H2, CH4, and CO2 storage to appropriate geological structures is a complex issue. It must take numerous conditions into account, including geological and reservoir conditions (Task 4.1.1) and physicochemical properties of the gases stored underground. Another important group of factors is legal aspects regulating the activity of underground gas storage sites, including storage safety. Other technical and economic (i.e. commerciality) factors have also to be considered during the design and operation of an underground gas storage site. Finally, potential synergies of H2/CO2/CH4 gas storage should also be examined (eg. Methanation of CO2/H2 mixtures).
Research Task 4.1.3: Petrophysical Changes and Reactive Flow Transport (UiS(L), ALL PARTNERS)
Changes in the petrophysics properties of reservoir rocks and effective hydrogen migration through porous media will be evaluated upon microbial activities and in the presence of water and cushion gas. The effect of capillary (residual) trapping and dissolution trapping will be analysed in this task. The aim is to evaluate how much of the injected hydrogen can be recovered through different injection and withdrawal cycles. Laboratory experiment will be executed in porous media (eg. sandstone, conglomerate) where hydrogen will be injected into rock samples containing water and microbes to evaluate the amount of hydrogen loss and adsorption. CT scanning, FTIR and wettability analysis will be done to assess the changes.
Task 4.2 hydrogen PROPERTIES affecting underground storage
To gain in depth understanding of the physico-chemical conditions of hydrogen gas storage.
Research Task 4.2.1: Reactivity and interactions between H2 and microorganisms (IFPEN(L), ALL PARTNERS)
Subsurface microorganisms can use H2 in their metabolism and thus may lead to a variety of undesired side effects such as H2 loss, H2S formation, methane formation, acid formation, clogging and corrosion. H2 reactivity questions the capacity of these systems to keep the quantity and composition of the gas injected during seasonal injection / withdrawal cycles. Task 4.2.1 focuses the characterization of hydrogenotrophic microbial populations in H2-rich deep environments using cultivation methods. These data enable the development of synthetic hydrogenotrophic microbial consortia and numerical simulations to study and model their activity. This task is currently conducted thanks to a master intern from Greece (Mrs. Elpida Maragkozoglou). This internship takes place from February to July 2024.
Task 4.2.2: Numerical simulations of H2 injection in porous media (IG/FORTH(L), ALL PARTNERS)
A first area of research concerns the role of hydrological circulations on a regional scale in the genesis and migration of H2. In this context, basin simulation is a fundamental tool to understand the migration of H2 from the bottom of the basin to the biosphere. Among other things, it is necessary to identify the preferential migration paths, taking into account occurrence of (active) faults, recognition of possible fault-patterns, the spatial distribution of porosities and permeabilities, and the pressure field, these different features depending on the geological history and the evolution of the basin. The results of the simulations can be compared with geochemical measurements made on samples taken in the field (geochemical rock analyses, isotopic measurements, noble gases).
A second area of research focuses on H2 storage at the reservoir scale. The injection and migration of H2 in the subsurface involve a large number of coupled processes: two-phase flow and transport, thermodynamic equilibria, dissolution and precipitation of minerals, kinetic biochemical reactions, dynamics of microbial populations, etc. Simulating these processes independently is possible today. However, the implementation of couplings in reasonable computing times remains a challenge. An effort still has to be made to propose a unified mathematical formulation for reactive multiphase flows and to develop suitable algorithms. This subject will be based on related topics developed at IFPEN such as the estimation of the risk of H2S production in reservoir simulation or on monitoring with dedicated tools developed at IFPEN like the Flair Suite.
Research Task 4.2.3: Thermodynamical aspects of H2 storage in porous medium (UiS(L), ALL PARTNERS)
In order to precisely size the processes where hydrogen is present, it is necessary to know the thermodynamic behavior of hydrogen in the different systems considered. This thermodynamic modeling will ultimately result in the development of an equation of state approach that can then be used in the various calculation simulators. The development of models is therefore based on the selection of appropriate equation of state for a given context and its parameterization. By using molecular simulation tools (Monte Carlo methods or molecular dynamics), it is possible to better understand the structure of fluid phases and thus propose the most predictive fluid model. Molecular simulation tools also make it possible to generate pseudo reference data under conditions that are difficult to access in the laboratory. Such information is then used at the macroscopic scale to define and parameterize the equations of state. Different contexts can be considered: hydrogen storage in salt/evaporitic cavern or aquifers for which phase equilibrium, fluid properties are investigated.
Task 4.3 A DECISION-MAKING tool for UNDERGROUN H2 STORAGE
Geological formations will be characterized for underground hydrogen storage. An integrated decision-making tool for the selection of potential hydrogen storage geological will also be developed.
Research Task 4.3.1: A decision making tool for the selection of potential hydrogen storage geological sites (IG/FORTH(L), ALL PARTNERS)
An integrated geochemical/mineralogical, bio-geochemical and thermodynamic numerical simulation model will be developed based on the results from RP4.1, RP4.2. Starting from an initial assessment, the proposed tool will be able to assess the performance of potential hydrogen storage geological sites for operators planning hydrogen storage facilities. The main outcomes of this tool will be the following:
- Identification of suitable geological sites for hydrogen storage and data acquisition requirements
- Assess cap rock integrity and seismic risk
- Reservoir description and characterization
- Assess subsurface distribution of injected fluids and log-term hydrogen-rock interactions
- Assess and mitigate subsurface risks of hydrogen operations
The potential to achieve cost-effective alternatives for hydrogen storage will be evaluated considering, among other, the possible financial problems over the lifetime, as well as the likelihood of technology scale and its application. The study will help the identification of the most promising options for geological hydrogen storage moving forward the definition of the overall business models.
Research Task 4.3.2: Potential sites for underground hydrogen storage in Greece (IG/FORTH(L), ALL PARTNERS)
Greece is a country with relatively good opportunities to manage the transition from the dependence on fossil fuels produced energy to an energy industry based on renewable energy sources (RES), supported by hydrogen as an energy carrier. This Task focuses on an analysis of the potential use of evaporites/salt caverns for hydrogen underground storage in Greece. The decision-making tool developed in Task 4.3.1 will be used.
Internships’ Research Activities
- Training on Dumux software
Partner Responsible: UiS
The PhD student and Scientific Associate of IG/FORTH, Efsevia Fragkou, visited the UiS under the supervision of Prof. Raoof Gholami (Department of Energy Resources) for 2 weeks, where she was trained in Dumux, an open-source simulator for flow and transport processes in porous media. Dumux is written in C++ and is based on the DUNE framework. It uses DUNE’s versatile grid interface, vector and matrix types, geometry and local basis functions, and linear solvers. Dumux has also integrated biochemical reactions in the code, pertaining to methanogens and sulphate reducing bacteria (SRBs). In the newest version of Dumux (3.7), SRBs are integrated but have yet to be activated. The user can change a number of microbial factors such as microbial growth rates, decay rates and yield. These features offer an advantage for reservoir simulation over commonly used Eclipse, and even though Dumux is still in its infancy, biochemical reactions are a crucial parameter to take into consideration in cases of Underground Gas Storage (UGS).
- Microbial processes in underground hydrogen storage
Partner Responsible: IFPEN
The PhD student and research associate of IG/FORTH, Georgia Charalampous, visited IFPEN under the supervision of Dr. Simon Poirier (Group leader of the Environmental Microbiology laboratory) for 2 weeks to participate in ongoing work on the characterization of hydrogenotrophic microbes using cultivation methods and molecular biology techniques (e.g. quantitative PCR). These groups of subsurface microorganisms affect the capacity of hydrogen storage systems in terms of quantity and composition of the gas injected during seasonal injection/withdrawal cycles. Kinetic parameters of hydrogenotrophic microbial consortia will be used in numerical simulations of flow and transport processes in Underground Hydrogen Storage (UHS) projects.